
Research
1. Biomolecular engineering: Directed evolution, high-throughput screening and DNA sequencing
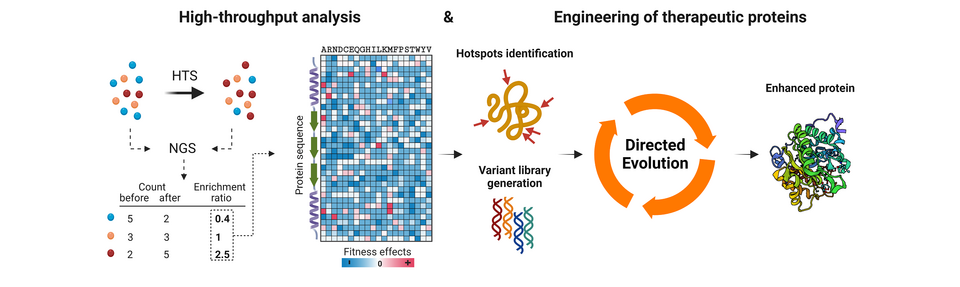
Keywords: High-throughput protein engineering, next-generation sequencing, machine learning, laboratory directed evolution, biologics, therapeutics, diagnostics
The pharmaceutical industry is rapidly transitioning from small molecule drugs to biologics. However, naturally occurring proteins frequently lack the requisite stability, specificity, developability, and function to be directly deployed as biopharmaceuticals. In order to enhance the molecular properties of therapeutic proteins, the Nash Lab develops novel methods to analyse sequence-structure-function relationships in a high-throughput manner. We develop screening techniques tailored to specific molecular functions that preserve genotype-phenotype linkage1–3. These methods are integrated with single-cell sorting and next-generation sequencing (NGS) in ultra-high-throughput (UHT) workflows, allowing us to investigate the impact of thousands of mutations on protein fitness4. Using the acquired insights, we construct custom combinatorial mutant libraries and employ laboratory directed evolution (DE), ultimately leading to the identification of improved protein variants5,6. Additionally, we harness computational tools and machine learning (ML) algorithms to aid the design of mutant libraries and conduct in-silico screening over an extensive combinatorial sequence space7. Our ultimate objective is to enhance protein therapeutics as well as gain a deeper understanding of the structural and functional mechanisms inherent in functional native proteins. This knowledge will significantly streamline protein engineering workflows, facilitating the successful development of biologics for clinical applications.
- Vanella, R. et al. Chem. Mater. (2019) doi: 10.1021/acs.chemmater.8b04348
- Lopez-Morales, J. et al. ACS Synth. Biol. 12, 419–431 (2023)
- Fernández De Santaella J. et al. Anal. Chem. 95, 7150–7157 (2023)
- Vanella, R. et al. BioRxiv 2023.02.24.529916 (2023) doi: 10.1101/2023.02.24.529916
- Vanella, R. et al. Biotechnol. Bioeng. (2019) doi: 10.1002/bit.27002
- Vanella, R. et al. Chem. Commun. 58, 2455–2467 (2022).
2. Mechanical biosystems: Deciphering mechanical properties of biomolecules
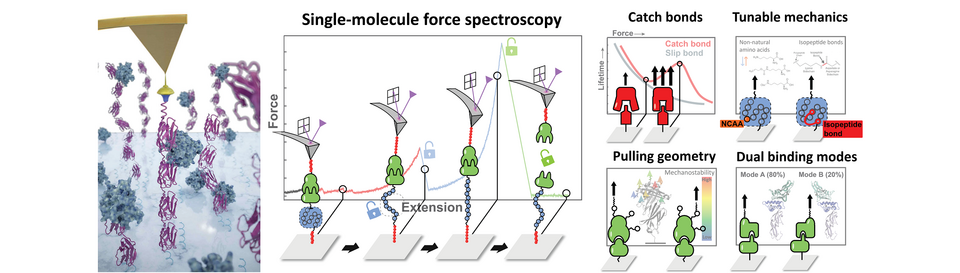
Keywords: Molecular biomechanics, atomic force microscopy, single-molecule force spectroscopy, single-molecule biophysics
Proteins can be significantly influenced by mechanical forces inside cells, tissues and organs in the body. These forces are essential to many biological processes, such as muscle contraction, blood coagulation, auditory perception, cell adhesion, and even bacterial pathogenesis. In fact, mechanical force can significantly modulate the function of proteins, for example by giving rise to new conformational states, revealing binding sites or altering enzymatic activity. Additionally, the effects of molecular forces on therapeutic proteins for medical applications is an evolving area of study.
The Nash Lab focuses on understanding the mechanical properties of proteins at the molecular level. We utilize the atomic force microscope (AFM) and high-throughput shear flow assays1 to measure the force resistance of molecules and molecular complexes. Using single-molecule force spectroscopy (SMFS), we quantify the energy landscapes that dictate protein folding/unfolding and receptor-ligand unbinding under applied force2-4. We also analyze and engineer protein-protein interactions that strengthen when force is applied (so-called "catch bonds"), which are essentially molecular analogs to a finger trap. We also study how differences in molecular loading geometry can influence the deformation and unbinding of individual biomolecules5-7. By harnessing single-molecule biophysical techniques, we seek to derive a comprehensive understanding of the mechanical properties of protein interactions. This knowledge will be pivotal for the strategic design and engineering of biological systems in the future.
- Santos, M. S., et al. Biophysical Reports, 2(1), 10035, (2022); doi:10.1016/j.bpr.2021.100035
- Yang, B., et al. Nano letters 20.12 (2020): 8940-8950; doi: 10.1021/acs.nanolett.0c04178
- Liu, Z., et al. Nature Communications 11, 4321 (2020); doi: 10.1038/s41467-020-18063-x
- Liu, H., et al. Nano Letters, 19(8): 5524-5529, (2019); doi: 10.1021/acs.nanolett.9b02062
- Liu, Z., et al. Nano Letters 22(1): 179–187, (2021); doi: 10.1021/acs.nanolett.1c03584
- Liu, H., et al. Angew. Chem. Int. Ed. (2023); doi: 10.1002/anie.202304136
- Yang, B., et al. ACS Nano, 18(46): 31912-31922, (2024); doi: https://doi.org/10.1021/acsnano.4c09220
3. Molecular sensors: Stimuli-responsive protein-based polymers
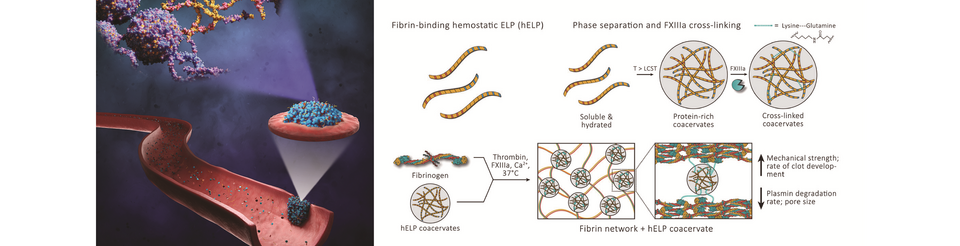
Keywords: Smart polymers, environmentally responsive proteins, elastin-like polypeptides
Stimuli-responsive or 'smart' polymers undergo dramatic conformational changes in response to slight variations in environmental conditions. Examples include small changes in pH, temperature, salt, and light that give rise to collapse or expansion of polymer chains in aqueous media. We work with both synthetic polymers and elastin-like polypeptides (repetitive protein polymers) that possess 'smart' properties. Our focus is on integrating smart materials into physiological processes to modulate biomolecular activity and related physiological processes in a predictable manner. For instance, in the coagulation cascade, by introducing recognition sites of FXIIIa, we managed to modulate the process of fibrinogen assembly in a temperature-responsive manner, thereby modulating the structure of the fibrin crosslinking network and improving biomechanical properties of blood clots. This advancement holds great promise for addressing severe pathological bleeding and coagulation disorders.
- Nash, Michael A. "Elastin-like Polypeptides: Protein-based Polymers for Biopharmaceutical Development: Medicinal Chemistry and Chemical Biology Highlights." Chimia 76.5 (2022): 478-478.
- Risser, Fanny, Joanan López-Morales, and Michael A. Nash. "Adhesive Virulence Factors of Staphylococcus aureus Resist Digestion by Coagulation Proteases Thrombin and Plasmin." ACS bio & med Chem Au 2.6 (2022): 586-599.
- Risser, Fanny, et al. "Engineered molecular therapeutics targeting fibrin and the coagulation system: a biophysical perspective." Biophysical reviews 14.2 (2022): 427-461.
- Urosev, Ivan, Joanan Lopez Morales, and Michael A. Nash. "Phase separation of intrinsically disordered protein polymers mechanically stiffens fibrin clots." Advanced Functional Materials 30.51 (2020): 2005245.